Research
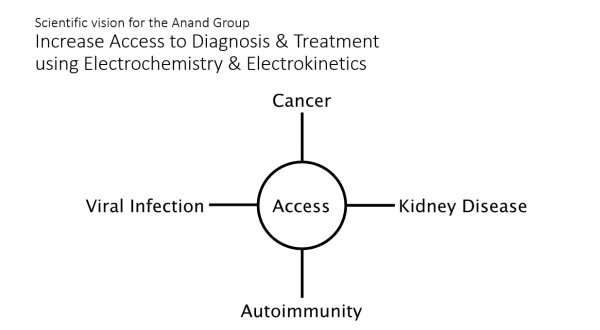
Research Overview
Research in the Anand group addresses critical needs in human health and advances sustainable energy technologies. Currently, the group is developing analytical methods and microscale devices to:
- Capture rare cell types to understand their role in cancer progression
- Analyze cells individually or in clusters to understand mechanisms of drug resistance and invasion in cancer
- Couple enrichment and electrochemical detection of disease biomarkers
- Enrich and remove waste components from dialysate fluid to improve treatment modalities available to patients with kidney disease
- Focus inflammatory markers in tear fluid for diagnosis of autoimmune disease
- Extract detailed electrochemical information from many reactions in parallel, without the need for multiple potentiostats, for multiplexed sensing and for screening electrocatalysts.
The contributions that we make to these fields leverage our expertise in bioanalysis, electrochemistry, microfluidics, and electrokinetics.
Cancer: Incomplete Diagnosis
Chemotherapy can select for subclonal populations of cells, thus creating refractory disease, resistant to the initial treatment. However, obtaining a complete picture of the genetic variability extant in tumor tissue can require the analysis of thousands of individually isolated cells. We are developing a dielectrophoresis (DEP)-based device with a high yield of single-cell capture in an array of microfluidic chambers for PCR-based analysis. The outcome of this research will be a clearer understanding of the role of clonal selection in cancer relapse.
Chemotherapy can select for subclonal populations of cells, thus creating refractory disease, resistant to the initial treatment. However, obtaining a complete picture of the genetic variability extant in tumor tissue can require the analysis of thousands of individually isolated cells. We are developing a dielectrophoresis (DEP)-based device with a high yield of single-cell capture in an array of microfluidic chambers for PCR-based analysis. The outcome of this research will be a clearer understanding of the role of clonal selection in cancer relapse.
In a key step to metastasis, cells migrate out of the primary tumor and into the bloodstream (extravasation). A fraction of these circulating tumor cells (CTCs) invade tissues (intravasation) distant from the primary site. We aim to uncover conditions that promote metastasis by developing microfluidic platforms for 1) high-throughput CTC isolation, 2) genetic analysis of individual CTCs, and 3) interrogation of the interaction of CTCs with on-chip tissue cultures that mimic blood vessel walls. The result of this research will be an understanding of the metastatic process leading to better-targeted treatments aimed at its prevention.
Due to the heterogeneity and extreme rarity of CTCs, the separation of CTCs from whole blood is challenging. Current separation techniques generally can be classified into immunoaffinity approaches, size-based filtration techniques, hydrodynamic chromatography, and DEP method. Separation based on DEP exhibits less bias when compared with the aforementioned approaches, and it leverages the unique composition, morphology and dielectric properties of CTCs. However, the relatively low throughput of current DEP devices is a critical issue that remains to be solved. Our lab has recently developed a method that employs an array of thousands of wireless electrodes to render microfluidic devices for DEP that are scalable along both x and y directions. Without ohmic contact to the individual array elements, capacitive charging of the electrical double layer (EDL) at opposing ends of each wireless electrode allows an AC electric field applied by only two driving electrodes to be transmitted across the entire device. The uniform DEP force exerted by the electric field gradient near each wireless electrode enables massively parallel and selective capture of CTCs. Inspired by the concept, we have successfully designed the parallel-channel device in which pockets are extruded at each side of microchannels. Using this design, single-cell capture can be readily achieved when the pocket dimensions were matched to those of the cells. We anticipate that the DEP approach presented in this work can provide a potential platform for high-throughput selective capture of rare cells and for single-cell analysis.
Kidney disease: Intermittent Dialysis
Between dialysis sessions, patients suffer from fluid retention and a gradual increase in blood concentration of excess salts and waste products such as urea. This process leads to both significant discomfort and eventual lung and cardiac damage. Existing devices require large fluid reservoirs and suffer from biofouling caused by direct contact between blood and membranes. We are developing a membrane-free reservoir-free device for reduction of excess fluid and urea using a series of electrokinetic processes such as contactless DEP and ion concentration polarization (ICP) (More details about ICP can be found in our review paper) . Our aim is to develop a wearable, battery-powered device.